E22 – Diamond Anvils and the Heart of Jupiter
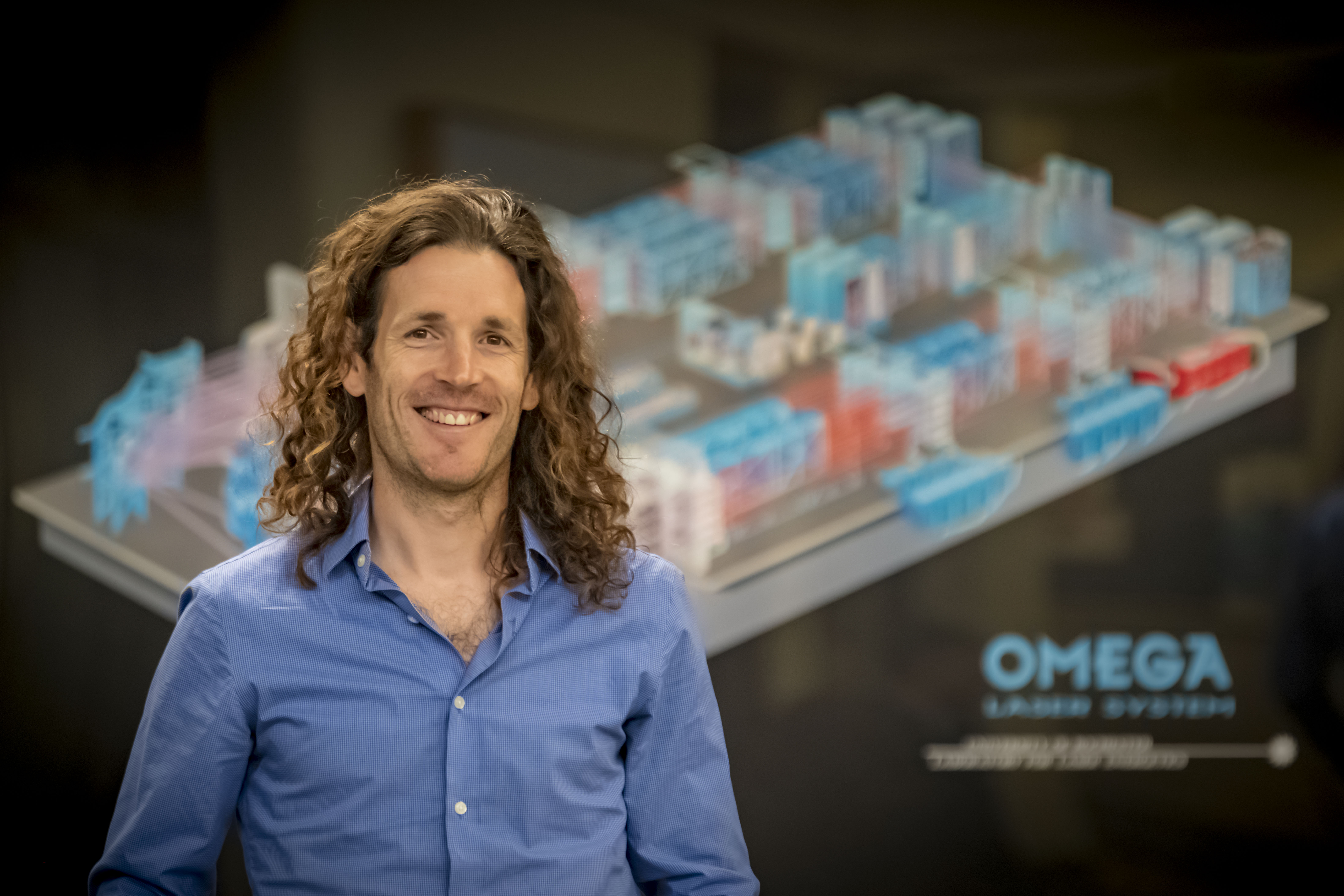
Marius Millot. Credit: E. Kowaluk/M. Millot/LLE/LLNL
Physicist Marius Millot investigates the intimate atomic worlds of elements in order to understand the inner secrets of the largest planets in our solar system. Jupiter and its fellow gas giants Uranus, Neptune and Saturn are comprised mostly of the lightest element, hydrogen, with a dab of helium, heavier elements, and more complex molecules. But researchers want to know what lurks at the planet’s core, under all that cloud. It isn’t clear that Jupiter has a surface to stand on that is at all like Earth’s—assuming anything could stand up under pressure approaching 100 million Earth atmospheres. That pressure may turn the depths into a hot, dense soup.
Millot can’t visit the Jupiter’s interior, but he can simulate some of its conditions here on Earth. As a researcher at Lawrence Livermore National Laboratory in California, he gets to play with giant lasers designed to explore atomic fusion. When the 192 lasers of at the National Ignition Facility focus on a single point, they can reproduce the unimaginably intense pressures in the interiors of planets, or even stars.
With diamond as an anvil, Millot and his colleagues have used the lasers to squeeze liquid hydrogen so tightly that it turns shiny, hinting that hydrogen near the center of the big planets may be a flowing metallic liquid, and perhaps the source of Jupiter’s mighty magnetic field. Millot explains how the center of Jupiter might just be a mushy blob in this episode of Third Pod from the Sun.
This episode was produced by Liza Lester and mixed by Kayla Surrey.
Transcript
Shane Hanlon: 00:00 Hi Nancy.
Nanci Bompey: 00:00 Hey Shane.
Shane Hanlon: 00:02 I wanted to know, do you remember the Mentos and soda thing?
Nanci Bompey: 00:07 No. I-
Shane Hanlon: 00:08 You have no idea?
Nanci Bompey: 00:09 What is the Mentos and soda thing?
Shane Hanlon: 00:10 So basically the idea is that you put Mentos into… People always do Coke, but some sort of chemical reaction happens that it results in the soda shooting up in the air and exploding. People would do it under pressure and explode bottles and all that type of thing.
Nanci Bompey: 00:25 Oh, I never did that.
Shane Hanlon: 00:27 No?
Nanci Bompey: 00:27 But we did do some interesting things in the microwave. Did you ever do any of those?
Shane Hanlon: 00:34 No. Who’s we?
Nanci Bompey: 00:35 No? Me and a babysitter I had. Which also made my parents very happy about that. Like a raisin. You put a raisin in the microwave.
Shane Hanlon: 00:43 Does it explode?
Nanci Bompey: 00:44 No, it puffs up real big. Yeah.
Shane Hanlon: 00:47 Oh.
Nanci Bompey: 00:47 Yeah. I think it may have eventually exploded.
Shane Hanlon: 00:50 And your parents didn’t like that very much?
Nanci Bompey: 00:50 Yeah, we did other … No. No.
Shane Hanlon: 00:57 Welcome to the American Geophysical Union’s podcast about the scientist and the methods behind the science. These are the stories you won’t read in the manuscript or hear in a lecture. I’m Shane Hanlon-
Nanci Bompey: 01:07 And I’m Nancy Bompey.
Shane Hanlon: 01:08 And this is Third Pod from the Sun. I have to say, I’ll never look at raisins the same way again.
Nanci Bompey: 01:21 Yeah.
Shane Hanlon: 01:21 Oh, we should do that in the microwaves here.
Nanci Bompey: 01:23 Oh, that would be really… They’d love us, I’m sure.
Shane Hanlon: 01:25 I’m sure our colleagues would love that. Anyways. Okay, so we’re talking about all of this and blowing things up and pressure. Well, I guess I don’t know. I’m going to ask Liza. So we’re going to bring in Liza Lester who is producer for this episode. Hey, Liza.
Liza Lester: 01:37 Hey guys.
Shane Hanlon: 01:39 So why are we talking about all this today?
Liza Lester: 01:42 So we’re talking about high pressure today. I talked with a high pressure physicist who is trying to recreate the conditions on the inside of Jupiter here on Earth, using lasers and diamonds.
Marius Millot: 01:56 My name is Marius Millot. I’m from France originally and now I work in Lawrence Livermore National Lab in California. And I mostly do experiment using large lasers to study matter at very high pressures and temperatures.
Liza Lester: 02:10 Okay. So Jupiter is mostly made of hydrogen, if you remember. The big gas planets.
Shane Hanlon: 02:14 I didn’t.
Nanci Bompey: 02:16 I know it’s not a rocky planet.
Shane Hanlon: 02:20 Okay, we’re getting derailed. I know it’s a gaseous planet.
Liza Lester: 02:22 Right? So it’s mostly gas. And this is one of the questions, is is it gas all the way down? What’s in the middle?
Nanci Bompey: 02:28 That’s a good question. Right?
Liza Lester: 02:30 And it matters because it matters for how planets behave and also how the solar system developed, as he’s going to talk about some more. One of the big questions is, what’s in the middle of things like Jupiter and how is it generating this big magnetic field that it has? Like Earth has a magnetic field and so does Jupiter, but some planets don’t, like Mars. And they have different magnetic fields for different reasons. But scientists think that it’s because there is molten metal of some kind moving in the inside of the planet. So it’s basically turning the planet into a big electric generator.
Shane Hanlon: 02:59 Hmm.
Nanci Bompey: 03:01 As in liquid?
Liza Lester: 03:02 Liquid. So on Earth it’s molten, it’s probably kind of like lava, right? It’s really hot rock that has metal content in it. And it’s moving around and it’s creating this flux. But then maybe a planet like Mars, it’s colder, it’s cooled down, so it doesn’t have this molten interior anymore. So then the question is, something like Jupiter, does it have this rocky thing on the inside or is it something different?
Shane Hanlon: 03:24 It doesn’t have rock, or it’s gas, right?
Liza Lester: 03:27 Does it have rock at all? Is maybe a question, right?
Shane Hanlon: 03:30 Okay.
Liza Lester: 03:30 Because it’s got to have some way. It has to have this motions of metal moving on the interior because it has a massive magnetosphere. Okay. So one of the things that Marius is looking at is what happens to hydrogen when you put it under lots and lots of pressure. That kind of pressure it’s under in the center of a big planet like Jupiter. And what he and his colleagues have found is that it does some weird stuff. One of those things is if you push on it enough, it can turn into a metallic liquid. Yeah.
Nanci Bompey: 03:58 Metallic liquid hydrogen.
Liza Lester: 04:02 Yeah. So it conducts electricity like a metal.
Shane Hanlon: 04:05 I had no idea.
Nanci Bompey: 04:05 No idea that could happen.
Liza Lester: 04:07 Right? And so first you press on it, maybe it becomes a liquid, but it’s more like a normal liquid. It’s not conducting, it’s not metallic. And if you keep going, they spied, you know, in these brief nanoseconds of these experiments that you could get this liquid that’s shiny like a metal. And so that’s important for understanding what might be inside Jupiter. Maybe it’s just this flowing metal hydrogen.
Nanci Bompey: 04:30 Now I’m pretty interested to hear how they do this, which I guess he will be telling us.
Liza Lester: 04:33 He does it with big lasers. He gets to play with big lasers and he’s going to tell us about that too.
Marius Millot: 04:41 Over there we have a big program where we trying to make nuclear fusion with big lasers, right? So we are trying to basically recreate a star on earth, right? So to tame nuclear fusion, where you take two hydrogen atoms and you bring them so close together that the nuclei of the hydrogen fuse and make a helium atom. So you take hydrogen and you make helium. And because of the E equals MC squared equation, you release energy doing that. And so that’s a very big scientific enterprise in which I’m a very, very small bit. Our colleagues do… Every week we do experiment where we reach 300 gigabar of pressures. So basically conditions just like inside the stars.
Marius Millot: 05:30 Now, most of my experiment and the experiment we were talking about, metallic hydrogen or studying the inside of Uranus and Neptune, those are megabar of pressure, millions of atmosphere. For planetary science it’s important because those technique are the only way that we can recreate the pressure and temperature that exists into a large planet. Jupiter, the pressure at the core is 80 megabars, 80 millions of atmospheres of pressure.
Marius Millot: 05:59 Over the years we’ve been trying to study different materials that are relevant for the planets, and we choose the most important ones. So we do hydrogen, we do helium, we do hydrogen, helium, and we do water because you know, the icy giant planets, or the gas giant planets. So laser is a light amplification by stimulated emission of radiation. So it’s basically a big amplifier of light. We take the energy from the electrical grid, we put it into big capacitor banks, then we charge a flashlight, which excite glass. And this glass then amplifies the laser light and this technology, this laser chamber, which fits in your laser pointer that you have, it’s about one centimeter.
Marius Millot: 06:49 Now for this laser, this takes three football fields long. The building is three football fields long. It’s 200 lasers, and each of them is the most powerful laser beam in the world. And they all fire at the same time and they all arrive on the target at the same moment. And with beautiful performance and optical shape and all the things that we want. We call it a target. We have this quasi-military jargon, right? We do a shot, and on the target. And so you have your target and you launch your laser energy onto it. And because it’s so much energy, the atoms and the molecules that form your target absorb this energy and become very, very hot. And because they become hot, they get ionized, it creates a plasma. And this plasma, it’s very hot and on one side it has a full density material. And on the other side it’s vacuum.
Marius Millot: 07:47 And so of course it expands where it can expand. And the recall of these plasma firing is what we use to compress the material. What’s exciting is that if you take water and you can press it to high pressure and temperature, just like Uranus and Neptune, you have completely different behaviors that the water that we know of everyday life. And the same for hydrogen, hydrogen, you take it, you compress it, it becomes opaque. If you put it into fluid and you compress it, it becomes liquid metal, which is just like liquid mercury, but very, very lightweight. More airy than lithium. So it’s completely defying your intuition and our intuition. But yet we do the experiment and that’s what we find and that’s what’s happening inside those planets.
Shane Hanlon: 08:39 Okay. So I have to ask, why do we care about this?
Liza Lester: 08:46 Because Jupiter is cool, Shane.
Shane Hanlon: 08:48 I know it’s cool. I know.
Liza Lester: 08:49 No, but a good question. Let’s let Marius explain.
Marius Millot: 08:53 A lot of people here at AGU are saying that if we want to understand how the planet formed, how it evolved in time and where it’s going to go and what’s happening with the climate, you really want to understand the Earth. If you want to understand the Earth today, you want to understand its history. If you want to understand the history, you want to know how the solar system formed, and if you want to know how the solar system formed, you need to study the giant planets. And so you go, you know, this staircase, and well, you need to know the giant planets, how do you model giant planets?
Marius Millot: 09:27 Well you look at it. You do astronomy, telescope, you say, “Okay, the radius is that much.” You see how it’s move around the sun. Okay? You get the mass and for the mass and the radius you get the density and you say, “Well, it’s pretty low so it’s got to be mostly hydrogen,” and then you can start to make model, how it came to form. There are basically two school of thought that how you formed this planet in the early solar system is, one, you have these gas nebula and in the early solar system you have a small rocky planet, protoplanet let’s say. And then this rocky planet starts to attract, by gravity, all the gas around it. And then you have a solid core of dense material that attracts all these hydrogen and helium and then makes this giant planet.
Marius Millot: 10:23 And therefore nowadays, if you believe that, the theory, there should be a big core, there should be a lot of metal and rocks inside Jupiter, right? And other people say, “No, you don’t need that. You can just have instability in the fluid and all of a sudden all the gas and the fluid hydrogen collapse and create this planet without the need for rocks and metals.” And one way to distinguish those theories is to look at Jupiter today and say, “Is there a core or isn’t it not a core?” And the way we do that, you know, it’s complicated. I’m doing a very, very little bit in in this, but it’s by sending spacecraft, right? So we just got very, very exciting data from the Juno Mission. The Juno Mission, there was a spacecraft orbiting around Jupiter, and measuring the gravity field by basically stopping every instrument on board to push. And then just looking at how gravity was affecting each moment.
Marius Millot: 11:34 Then you get a gravity field. So then you get the mass distribution inside the planet. Then you also measure the magnetic field. So then you get basically the flows of conducting fluid inside the planet. And then our colleagues, they take this data and say, “Okay, what’s inside Jupiter now?” To do that, to go from the magnetic field and the gravity field measurement to a model of the planet, you need to know how hydrogen compresses as a function of pressure and temperature. And you need to know the electrical conductivity of hydrogen as a function of pressure and temperature and the viscosity and the thermal connectivity and all these properties. And of course you cannot measure everything, but we can do a few measurements In particular we can measure pressure density, compressibility as a function of pressure and temperature, at the condition inside those planets. We can also measure the electrical conductivity by looking at optical properties. And in particular the moment where hydrogen goes from molecular fluid to a metallic fluid.
Marius Millot: 12:42 So with pressure and temperature, you take hydrogen and if you were to fly inside Jupiter, you go from the atmosphere, it’s a molecular gas. You can’t see it. But if you go down and down, as the pressure increases, the atom gets closer and closer together, the molecule gets closer and closer together, to the point that you start to break the molecule and release free electrons. And so basically you’re breaking the bonds and making a metal at the same time in the fluid phase. And that’s something we can do experiment on. We can show that it actually exists. It’s true. Hard to make. And then our colleagues make models of how these changes with pressure and temperature and then the other colleagues use that to model the planet.
Marius Millot: 13:32 Then, we can do fantastic experiment. And that’s what I find exciting is that we have these great facilities, really big facilities. And of course there is a lot of preparation in advance, right? But the day you do your experiment, then you have a team of people that are working here just to make your experiment happen. And if you thought it well, if you did your homework, then you gave them all the instructions to make the experiment that you want to happen. So all the things will happen and you will compress the material the way you want it to compress. And then you will trigger all the different measurement instruments at the right time and looking at the right position with the right settings to make our measurements. Because, of course, once the shockwave has passed your sample, everything decompresses, everything is vaporized and you have to wait and the laser cools down, and then put another target if you’re lucky and have the right to have another shot.
Liza Lester: 14:36 How often do you get to do your experiment?
Marius Millot: 14:39 So it depends a little bit on how high in pressure and how big a laser we need. The National Ignition Facility in Livermore fires about 400 times a year. So each person doesn’t do a lot of experiment on each laser every day. Typically you have a few per year on the National Ignition Facility. But at the OMEGA laser, which is in Rochester, New York, it’s a smaller scale laser. It fires about 15 times in a day, and they fire it three days a week. And so there, we go there pretty much every once every two months or something like that. And then we do what we call a campaign. So we do either half a day or a day of experiment. And so we do between six and 15 experiment. There’s a lot of preparation, so you really want to make the most of these facilities.
Marius Millot: 15:30 We usually have several people working on the same experiment so that we can send ideas to each other and say, “Yeah, I don’t agree. Yes, I agree. What do you think is happening? Well, I think this is happening.”
Liza Lester: 15:42 What does it feel like when experiment day is approaching?
Marius Millot: 15:45 So actually last week I was over there in Rochester, New York and we did a set of experiment. I’ve been doing those experiment for about eight years so it’s routine now. You go there, you have these big laser. Yeah, we have all these team of people and yes, there’s a shot director counting, making the countdown and saying, you know, all the personnel have to evacuate and we’re about to charge the laser, and then making the countdown for the charging of the laser and the countdown for the shot.
Speaker 5: 16:14 Ten, nine, eight, seven [crosstalk 00:16:17]
Marius Millot: 16:17 And then you see the instruments having the triggers, and saying, yeah, acquisition ready, and then you shoot.
Speaker 5: 16:24 Shot number 92425 [crosstalk 00:16:29].
Marius Millot: 16:29 But every time you shoot… And we use CCD cameras for our diagnostics, so we are lucky now that those acquire pretty fast. And then we get the data on the computers in the control room pretty quickly, like a few seconds after the shot. But those five seconds we have to wait between the moment that the show director announced the shot, and then the moment that the image refreshes, we’re like, “Okay, what’s happening, what’s happening?” And each time we are very nervous, and then we see the result.
Marius Millot: 16:56 And our experiment, most of them we can tell right away if it worked or not. We have this optical diagnostic that really tell us a lot of things about the experiment. And so you see the data, you’re like, “Oh yeah,” “Oh no, it didn’t work. We have to try another one.” But sometimes when it works, it’s really exciting. But every time we shoot the laser, we are very nervous and we are really hoping that we didn’t make the mistake. And sometime we do make mistakes. But yeah, that’s what’s exciting about those experiments.
Shane Hanlon: 17:32 So hearing this, literally the only thing I can think of is Austin Powers. It’s like Dr Evil, like, “Sharks with fricking laser beams attached to their heads.”
Nanci Bompey: 17:42 Right. Right, right. No, no, no.
Shane Hanlon: 17:44 That’s all I got.
Nanci Bompey: 17:44 But these are not sharks with laser beams.
Shane Hanlon: 17:46 No.
Nanci Bompey: 17:47 Not here in this lab.
Shane Hanlon: 17:48 No. Not sharks nor shooting at Austin Powers.
Nanci Bompey: 17:50 No. That would be interesting [crosstalk 00:17:53] experiment.
Liza Lester: 17:53 We hope not.
Nanci Bompey: 17:53 But it’s not. So what are they shooting the lasers at in their lab?
Liza Lester: 17:59 So they’re trying to shoot at their sample, which is very small, and to contain it, they have this thing called a diamond anvil. Cool, right?
Marius Millot: 18:08 You have to remember that pressure is force divided by area. Right? So if you are able to apply a force on a large surface and transmit this force to a small area, then you multiply the pressure, right? And the best material that we have to do these kinds of things is diamond because it’s the hardest material we have on hand. And it’s also great because it’s transparent to the optical light. It’s pretty transparent to X-rays. It’s pretty transparent to neutrons. So you can take your sample and what you do is you take two gems of diamond and you flatten just the top of the tip. You make two small surface, typically a hundred micron or 500 micron in diameter. And where the big table is like one, two, three millimeters in diameter, right?
Marius Millot: 19:00 So you take these two inverse pyramids, let’s say of a diamond with a flat at the top, you put them in a position and then you put your sample in between and then you push on the big surface and now you multiply your pressure. So then you can reach very high pressure with this cell. And of course the two diamonds are playing the role of the anvil. So it’s a diamond anvil cell. And you squeeze and you have your little sample that is being compressed to very high pressures.
Liza Lester: 19:30 This will sound dumb, but why doesn’t it squeeze out the sides?
Marius Millot: 19:34 So actually what we do is we put a gasket, so we put a metallic gasket. So what you do is you put a metal foil in between your diamond in which you drill a little hole that is smaller than the size of the diamond. So then when you compress, you actually pressing and making the metal flow. And that’s your gasket. It’s not like a plastic gasket, it’s not a silicon gasket, it’s metal gasket. And actually in some experiment, we push so hard on the metal that the metal really flows and that make your experiment fail. So that’s one thing that people are very good at, is designing ways to make these metallic gasket contain your material. Yeah. So you push on from the top and the bottom, and you have this metal resisting in the equator, let’s say. And so that’s what you have, this pressure volume.
Marius Millot: 20:34 What’s interesting is that the device was invented in Rochester, in New York. 60 years ago, a researcher named Dave Mao came to the Geophysical Lab here in Washington DC, or the Carnegie Institution For Science, and he was working in Rochester and he came here for a postdoc and he brought the diamond cell, which was very young, very new device, crazy instrument. And he say, “I’m going to use it this to study mineral physics. I’m going to use a diamond cell to study what’s happening inside the Earth. Let’s look at the pressure. We know if you go in the mantle, it’s several gigapascal. If you go again to the core-mantle boundary it’s 100 gigapascal. Let’s put minerals in the pressure cell and see what happens.”
Marius Millot: 21:24 And so he basically launched the whole field of mineral physics and the high pressure and it all started right here, right here, like three miles away North from here.
Liza Lester: 21:33 Is this a new idea, that hydrogen can become not only a liquid but electrically conductive under high enough pressure?
Marius Millot: 21:40 So it’s not really a a new idea, right? It’s been around for a while. And actually I think people have theorized that and proposed and used some measurement to say that there is a magnetic field around Jupiter. I think the first measurement from the Earth were 1955. And see, if you have a strong magnetic field around Jupiter, the only way can come to be be generated is to magnet to hydrodynamic, right? So basically dynamo effect, just like in the Earth, right? So it’s a turbulent flow of a conducting fluid, right?
Marius Millot: 22:21 You have Jupiter, we know its density, it’s mostly hydrogen and there is a big magnetic field. So there is not too many options as to what’s conducting, right? It has to be the hydrogen conducting in the fluid phase. And then in the ’70s they send this first spacecraft that we actually probe the magnetic field in situ and say, “Yes there is a magnetic field.” And then more people did more measurements. And now we have very, very accurate measurements.
Marius Millot: 22:50 So let’s say we have the astronomy observation that there is something conducting in this planet and in Saturn as well. And in the lab, people have theorized, in the 1930s, that if you take hydrogen at very low temperature, just in the solid phase, you take hydrogen’s molecular system, if you compress it to high density, you reach a point where this density is high enough that you’re going to break the bonds and delocalize the electron. Basically it’s a quantum mechanic effect that the electrons are localized in the molecule, but that has a cost. And so if you bring these molecule too close to each other, at some point the systems prefers to be in a state where those electrons are shared across the crystal in a delocalized state, and that’s a metal.
Marius Millot: 23:47 And so there was this prediction, 1930s, you take hydrogen, you squeeze it enough, it will become a metal. And then people have done a lot of experiments on this. And what’s interesting is that actually it’s not just hydrogen. If you take oxygen, if you take sulfur, if you take pretty much a lot of things that are not metals at any other condition, if you squeeze them enough… The enough is an important thing. Some things we have been able to show that it does turn into a metal, some material still we are waiting for the confirmation, but a lot of things that are insulating, if you squeeze them enough, you can turn them into metals.
Marius Millot: 24:34 What’s new and what’s difficult and where we are making progress now is that we can study these phenomena with very, very high accuracy. We can really test the theories and the numerical simulation with very, very high precision.
Shane Hanlon: 24:54 All right, so he’s seen metallic liquid hydrogen in the lab, right?
Liza Lester: 24:59 They’ve seen it.
Shane Hanlon: 24:59 That’s pretty cool.
Liza Lester: 25:00 Right?
Shane Hanlon: 25:01 Yeah.
Liza Lester: 25:01 Yeah. They’re not the first to see it, in fact, actually it was seen at Sandia National Labs in Albuquerque, using a different machine, and they repeated the experiment and there were some discrepancies.
Shane Hanlon: 25:12 That’s science.
Liza Lester: 25:13 Science, you know, they were trying to figure out, well, you know, the experiments aren’t wrong, so how does it fit together?
Marius Millot: 25:19 Yeah, yeah. They are pretty interesting discussions, let’s put it that way. About four years ago, our colleagues at the Sandia National Lab in Albuquerque made an experiment. They use a different source to make the pressure. They use a magnetic pulse power source, but it’s very similar in nature. It’s very fast compression in a few hundreds of nanoseconds in their case. And they compressed this hydrogen, and then they looked with optical diagnostic and they saw that it was first, they were compressing it, it was turning into an opaque material, so it basically becoming black. And then as it kept compressing, of course, all of that is very fast and we make the story afterwards. They kept compressing and then they found that it turned into a very highly reflecting system. So it looks really like a metal, really like a shiny metal.
Liza Lester: 26:13 Shiny?
Marius Millot: 26:13 Exactly. But what was very surprising that the pressure at which they found this transition into the metal state was much higher than most theory predictions, and also much higher than other experiments using diamond cells. And so we started to do an experimental campaign to look at this problem. And basically what we did is we did very similar experiment. We compressed hydrogen in the fluid phase with our large laser. And the diagnostic is very similar to what our colleague did. The main difference is that our experiment is 10 times faster, 10 to a hundred times faster. And what we found is that hydrogen does turn from transparent to opaque and opaque to reflecting, but we found a transition at much lower pressure.
Marius Millot: 27:02 And so, of course, colleague doing theories, they say, “Oh yeah, it’s great. It agrees with our theory.” You know, everything is pretty tricky. Right? I think ultimately all the experiments are correct. It’s not that one experiment is incorrect and the other is correct. We need to understand all the observations with one correct model for what’s happening, how to understand those experiments, before we give the package and give it to our colleagues and say, “This is what’s happening in the hydrogen metalization.”
Liza Lester: 27:36 So before you can go and say, “This is what’s happening on Jupiter,” you need to understand a little better what you are seeing in your laboratory experiments.
Marius Millot: 27:44 Yeah.
Liza Lester: 27:45 What do you think is the answer? Do you think Jupiter has this liquid metallic core or is it solid like we learned when we were in grade school?
Marius Millot: 27:54 Yeah. With the current knowledge, it seems that the Juno results tend to suggest that there is indeed heavy elements in the center of Jupiter. But what seems to be to be indicated is that it’s not maybe just like a very defined core surrounded by metallic hydrogen, but perhaps is more metals and rock at the center and slowly, gradually evolving to metallic hydrogen, metallic fluid hydrogen around it. Because colleagues have done predictions, I’ve looked in simulations, our colleagues have looked at the chemistry between metallic hydrogen and rocks, so you take metallic hydrogen, you mix it with rocks, do you dissolve the rocks or do you have a mixed system? Because that’s very important. In your planets, you have millions of years, billions of years and you need to know that. Because if they are happy to mix, if they are resolving, interacting with each other, then there’s no way you’re going to have a very well-defined core. And so our colleagues doing simulation say, “Well it may be happening that the core is being eroded over time by the metallic hydrogen, by the convection in the in metallic hydrogen.”
Marius Millot: 29:32 The data from Juno seems to be in agreement with that hypothesis, that metallic hydrogen would react with whatever is in the core and make a very gradual, mushy blob at the center, not like very well-defined core surrounded by an atmosphere. Basically there is no surface around the core of Jupiter. That’s a proposed understanding. But of course I’m an experimentalist, so what I really want to do is do the experiment, take metallic hydrogen and rocks and metals and see if we can show that it does happen. But that’s a little bit more complicated, so we’ll have to wait for that one.
Nanci Bompey: 30:21 So my profound thought about this-
Shane Hanlon: 30:25 Hey, I’m in full support of it.
Nanci Bompey: 30:27 … is, we know a lot of stuff about things, but we don’t know a lot of stuff about things.
Shane Hanlon: 30:34 Yeah. I mean, I’m with you. And sometimes to learn stuff, you get to use frickin’ lasers.
Nanci Bompey: 30:42 Yeah.
Shane Hanlon: 30:43 How cool was that?
Nanci Bompey: 30:44 It really is pretty cool.
Shane Hanlon: 30:47 Yeah, I’m on board with this.
Nanci Bompey: 30:49 Yeah.
Shane Hanlon: 30:53 All right. Okay folks. Well, that’s all from Third Pod from the Sun.
Nanci Bompey: 30:56 Thanks so much to Liza for bringing us this story and to Marius for sharing his work with us.
Shane Hanlon: 31:02 This podcast was produced by Liza Lester and mixed by Kayla Surrey.
Nanci Bompey: 31:06 We would love to hear your thoughts. Please rate and review us, and you can always find new episodes wherever you get your podcasts or at ThirdPodfromtheSun.com.
Shane Hanlon: 31:15 Thanks all. And we’ll see you next time.